SARS-CoV-2 and COVID-19: From Crisis to Solution
Author Affiliations: World Health Network
Corresponding author: Spela, Salamon, salamon.spela.md@gmail.com
Abstract
The global impact of the COVID-19 pandemic persists, causing significant harm. Extensive evidence indicates that even mild infections and reinfections can result in symptomatic and subclinical health damage, disability, and persistent infection. Vascular impacts, neurotropism, and immune dysregulation lead to impaired organ function, increased morbidity and mortality, compromised work productivity, and a decline in overall health and quality of life. The uncontrolled spread of the virus is accelerating its evolution, outpacing the effectiveness of vaccines, treatments, and immune system adaptation. This preventable disease and others magnified by immune dysfunction are driving staff shortages, supply chain disruptions, and overwhelming healthcare systems. Despite the dire nature of the current conditions, knowledge and means are present to solve these problems. We present a science-based strategy for confronting the ongoing pandemic, including reducing airborne transmission through clean indoor air programs comparable with historical clean water programs. Public and professional education on the implications of repeated SARS-CoV-2 infections and utilizing known preventive measures can dramatically reduce transmission, which in turn reduces the rate of new variant introduction and strengthens the effectiveness of vaccines and treatments. It is essential to restore the prioritization of health and safety in healthcare and society.
Introduction
While many public health officials continue to focus on acute respiratory manifestations, COVID-19 is primarily a vascular disease with the ability to cause widespread multisystem damage. We have gained considerable knowledge regarding COVID-19 since the emergence of SARS-CoV-2 in late 2019. While this virus primarily infects through the respiratory route via inhalation of infectious aerosols [1], it primarily causes a systemic (micro)thrombotic endotheliitis [2-4] and directly attacks also the immune [5-7] and nervous [8-10] systems. It has become clear that the acute disease stage, whether severe, mild or even asymptomatic, is often just the initial phase of persistent infectious disease, which can take up residence in many organ systems [11-13] and commonly cause ongoing health issues [14]. The long-term effects of COVID-19 on both infected individuals and society are not yet fully understood, however they are severe at the individual and population levels, and almost all investigated health risks continue to grow with each new reinfection in all age groups [15-17]. The increasingly detrimental effects of repeated infections were recently summarized in a Canadian study showing the long-term effects of the virus on lasting symptoms (Fig.1). In the general population, the odds of developing symptomatic long COVID were about 15 % after the first infection, 25 % after the second and 38 % after the third infection. Half the patients reported no improvement of symptoms over time and two thirds did not receive adequate medical care [18]. Crucially, while vaccination decreases acute stage hospitalizations and deaths [19], vaccination alone [20] only partially prevents post-acute disease manifestations and long-term health complications even in the young and previously healthy [21,22] and cannot reliably prevent infection or slow down viral evolution [23]. However, our current public health messaging and responses fail to adequately address these facts. The absence of accurate information creates room for conspiracy theories and misinformation.
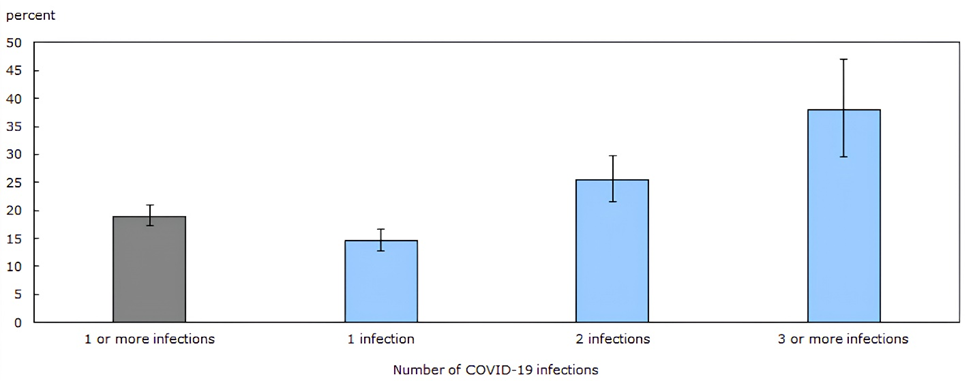
The Disease
Multisystem and organ damage
Numerous studies have shown that SARS-CoV-2 infections and reinfections lead to serious long-term pathology, both symptomatic and subclinical, in all age groups [24,25]. Even acutely mild or initially asymptomatic cases can result in lasting health damage, with or without persistent or apparent symptoms [26-30] including in children [17,31-34] and in two thirds of patients with symptomatic long COVID, organ impairment was detected [35]. As a primarily vascular virus, it damages multiple organs and body systems [35,36]. SARS-CoV-2 spreads through the airways, but chiefly infects and damages the blood vessels [37-39] causing excessive coagulation resulting in both large and microscopic thrombosis [40-42] It directly infects and ignites inflammation in atherosclerotic plaques regardless of their severity [43]. Resulting arterial stiffness after mild initial disease may worsen in a progressive, degenerative manner [44]. Consequently, heart disease risks soar for at least a year following infection [45-47]. The risk of dyslipidemia [48] and hypertension [49] is elevated. The infection can weaken [50], exhaust [51] and damage [5, 52] the immune system, including by direct T-cell attack [5]. Immune dysfunction is profound and multifaceted in COVID-19 [6,7,53,54]: for example, monocytes are reprogrammed to cause blood coagulation instead of defending against infections [6]. T cells, B cells, dendritic cells, platelets, and monocytes are also affected [55]. This immune dysfunction leaves patients vulnerable to other infections [5,51,56-58] and likely also cancers [59-63]. For example, the risk of other viral infections following COVID-19 increases more than four-fold, which was already known in 2021 [64]. On the other hand, a COVID-19 infection can also turn the immune system against its own tissues and trigger autoimmune conditions [65-68]. It also affects many other organs and systems, such as pulmonary function and the development of pulmonary fibrosis [69,70]. It can damage the kidneys and cause a significant long-term decrease of kidney function [71], infect and damage the liver [72], increase the risk of new onset diabetes and disrupt glycometabolic control [73-75] invade, damage and persist in the brain, impairing its metabolism [4,9,76-79] and compromise neuronal activity by causing neurons to fuse [80]. The brain injury caused by infection is associated with the development and acceleration of dementias [81,82] cognitive decline [83-86] hormonal disturbances [87], depression, anxiety, behavioral changes and substance abuse which may be falsely attributed to other causes [88,89]. It threatens human fertility and sexuality in various ways, including by directly infecting the gonads [90,91] increasing the risk of hypogonadism [92] and erectile dysfunction [93], negatively affecting sperm quality parameters [94], crossing the placental barrier, infecting and damaging the placenta [95] and the fetus potentially several months following birth [96,97] and is associated with increased risk of preeclampsia, fetal loss, and preterm birth [98]. Infection can cause acceleration of biological aging and telomere shortening [99], impair mitochondria [100], damage DNA [101,102] and chromatin [103]. The virus can take up residence in body tissues for months after acute infection [11,12,104-106] which can cause ongoing harm.
Individual, population and hybrid immunity
The concept of herd immunity, whether from vaccination, infection, or a combination of them (“hybrid” immunity) has been widely discussed for SARS-CoV-2 but only scientifically applies to an infectious disease when persistent immunity of an adequately high percentage of the population leads to prevention of widespread ongoing infection. Since transmission continues at a high level it is apparent that it does not apply to SARS-CoV-2 under current conditions [107]. Specific acquired immunity obtained from either vaccination or infection is temporary both due to waning of the immune response and due to the evolutionary introduction of new variants which evade prior immunity [52,108].The rate of mutation and new variant introduction are linked to the rates of infection which is high across the population globally. Each new reinfection facilitates evolution of immune escape [52,109] so that acquired immunity does not provide sufficient protection from disease spread and its impacts [15]. Specific studies of hybrid immunity have shown immunity against infection to be less durable for Omicron than for Delta [110] and to wane more rapidly than protection against severe disease and hospitalization [111]. In addition, past infection can increase susceptibility to COVID-19 reinfections [112,113] which are becoming increasingly common [114]. Facing a constantly mutating, immune-disrupting virus, herd immunity is unattainable. Due to uncontrolled viral spread [115], it continues to evolve to outmaneuver our immune systems [116]. The only means to limit the rate of viral evolution is to control the spread of the virus .
Pathogenicity, acute death rates and life expectancy
During the first week of the acute disease stage, when infectiousness is highest [117-119] mortality and severe symptom burden is typically low. This does not indicate selective pressure toward decreased pathogenicity, especially for post-acute disease. The basics of viral evolution teach us that each replication is a new chance for mutation, therefore the extent of spread is one of the main determinants of the new variant emergence rate [120]. These new variants are subject to evolutionary pressure, which constantly pushes them toward higher infectivity and better immune evasion [108,115] as well as resistance to treatments and vaccines, evidenced by the history of such variants [121]. Previous infection may elevate the risk of severe acute stage illness following subsequent reinfections [122,123]. Therefore, the concept of selective pressure toward decreased pathogenicity, especially post-acute pathogenicity, is not applicable to COVID-19 based on general evolutionary principles or observed variant history, and expecting the virus to spontaneously mutate into a less pathogenic form is not supported by science. Conversely, reinfections are not necessarily “milder”, even in the acute stage [124]. Each additional reinfection, even if initially “mild”, contributes to long-term symptoms and health risks in adults [15] and children [17,32]. While many are reassured by decreasing acute stage death rates [125], COVID-19 continues to be among the most commonly identified causes of death in spite of limited surveillance [126]. Acute disease severity reductions are largely due to improvements in acute disease stage treatments and in part due to short-term immunity against severe acute stage disease which wanes increasingly rapidly since the emergence of Omicron lineages [127-129]. Nevertheless, acute death rates still remain five times higher than for those hospitalized with influenza [127]. In addition, there has been a sharp decline in global life expectancy since 2020 and countries with most proactive infection control measures have largely avoided it [130].
Chronic illness, symptom burden and disability
After only one infection, 10-30 % of the non-hospitalized and 50-70% of the hospitalized patients experience symptomatic post-acute sequelae of COVID, also known as PACS (post-acute COVID syndrome or post-acute COVID-19 sequelae), PASC (post-acute sequelae of COVID-19), PCC (post-COVID condition) or PCS (post-COVID syndrome), commonly called long COVID [131]. Al-Aly et. al. calculated that a SARS-CoV-2 infection led to over 80 disability-adjusted life years (DALYs) lost per 1000 people who weren’t hospitalized (0.08 years of life per person), and more than 640 DALYs per 1000 people who were (0.64 years of life per person). Cancer and heart disease each have DALYs of about 50 [26]. While everyone is at risk, it is important to note that studies that focus on healthcare and frontline workers who are generally unable to work from home show they are facing worsening post-acute COVID-19 symptoms and loss of income due to inability to work [132,133] along with general burnout and post-traumatic stress, exacerbating workforce shortages [134,135]. This leads to a shortage of care, not only for COVID-19 patients, but for everyone who is sick or injured. Therefore, the potential consequences of uncontrolled spread go far beyond immediate impacts on hospital capacity and acute mortality. It has also been shown that greater harms are experienced by people of lower socioeconomic status, ethnic minorities, marginalized communities [136,137] and women [138,139] who are affected at a greater rate by post-acute disease. Stigma and discrimination are not limited to those who have prior socio-economic disadvantages, as they can affect post-acute COVID-19 patients in general [140], which expands the scope of the problem to other socio-economic groups and confounds its accurate assessment.
Comparison to other pathogens
A review of existing scientific literature clearly reveals that comparisons to “other respiratory infections” are inaccurate. While SARS-CoV-2 primarily spreads through the airborne route [141], it is biologically a systemic, immunotropic, neurotropic, and thrombogenic endotheliitis, with invasion into many types of tissues, and high potential for persistent viral infection and chronic viral disease [11,13,105,142] The harm caused by COVID-19 infections is not similar to influenza, rhinoviruses, or other mainly acute and self-limiting respiratory infections, even if these can sometimes also cause long-term effects. None have the scope of effects as listed above. SARS-CoV-2 was categorized as a Biosafety level 3 pathogen together with Mycobacterium tuberculosis (Tuberculosis), Yersinia pestis (bubonic plague), Yellow Fever virus, and Avian Influenza virus [143] and its access and handling in the laboratory setting remain strictly controlled due to its airborne spread and high virulence, yet unlike other diseases in its category, it has been allowed to spread, replicate, and mutate freely in the population. Seasonal respiratory infections such as Influenza, Parainfluenza, Rhinoviruses, Adenoviruses and similar are conversely Biosafety level 2 pathogens. In comparing COVID-19 with other diseases, we clearly see that it affects a greater number of systems due to its vascular mode of attack. In terms of pathology, other comparable diseases with potential for chronic persistent infection and organ damage include HCV / Hepatitis C with damage and increased cancer risk to the liver, group A Streptococcus / Scarlet fever, damaging the heart valves, kidneys, joints and brain, HIV/AIDS particularly damaging the immune system allowing opportunistic infections and cancers [144], and, H. pylori causing ongoing stomach damage and increasing stomach cancer risk [145]. Damage to the immune system by SARS-CoV-2 has both differences and striking similarities to long-term outcomes of HIV infection [50,144,146,147] and both are recognized as main causes of lymphocytopenia [148]. Importantly, these other debilitating chronic diseases also tend to have an influenza-like acute disease onset, but we differentiate the flu-like symptoms of initial HIV and HCV infection from the chronic conditions of AIDS and Hepatitis C, and recognize their causal connection.
Broader impact on individuals and society, and mental health impacts
The consequences of organ and systemic damage have only begun to be considered. Individuals who experience long-term health issues may be unable to work [149] or require long-term medical care [150], leading to decreased productivity and increased healthcare costs. Polls and scientific analyses from across the globe tell a disheartening story of the reach and duration of symptomatic long COVID after acute disease of any severity [140,151-154]. About one third of patients are not getting better, one year [153], two years [155,156], or longer after initial infection. It remains unknown if they will ever recover, and doctors have few effective, evidence-based treatments to offer [131]. Many others grapple with seemingly unrelated health issues because they were never tested for COVID-19 [157]. Moreover, even when confirmed, physicians struggle to connect many symptoms to this still relatively novel and highly complex disease. Instead of being offered the few known evidence-based treatments [131] patients often face uninformed clinical care [158] including exposure to unsafe conditions in healthcare settings [159]. Infections directly and indirectly harm the nervous system and are associated with mental health issues in children [160] and adults alike [31]. The ability of individuals to comprehend and effectively respond to individual challenges may be diminished by the direct neurological damage from widespread and often undiagnosed “mild” SARS-CoV-2 infections [79,85]. The observed harm to ability to think, remember, and make decisions implies we are only beginning to understand the impact of COVID-19 induced brain damage on interpersonal and intergroup violence, traffic safety, addictive behavior, the quality of intellectual and specialist work, scientific advancement, etc. Children who experience trauma and stress during the pandemic, such as losing their parents or caretakers [161], or suffering the physical health effects of infections and reinfections [17], may also develop lifelong mental health issues. The mental health impacts of the ongoing uncontrolled pandemic are growing, because the risks of serious neurological and psychiatric outcomes persist for two years after infection and beyond, and have not changed between the delta and omicron waves [162].
Public health reporting and messaging
It is crucial for informed consent as well as for public health precaution adherence to provide the public with accurate information about the risks of infections, the nature of airborne transmission and effective protective measures. As trusted authorities, healthcare providers must be well-informed and honest about the consequences of repeated infections, the (limited) effectiveness of vaccines and treatments, and the airborne nature of disease transmission and its prevention. Conversely, throughout the pandemic, optimistic speculations and outright misinformation [163] even from professional and official sources have been pervasive. One example is transmission in schools. Repeated claims that schools are safe and children do not spread the disease were never scientifically justified, and are well established to be invalid, with studies showing that more than 70% of infections in the community originate from children [164]. Outdated clinical definitions of COVID-19 based on the acute respiratory aspect of the disease from 2020 continue to be used to count COVID-19 hospitalizations and deaths worldwide, which makes these figures unreliable in trying to estimate the realistic impacts of infections. The recent increase of sudden unexplained deaths of previously healthy people has been directly linked to the disease, not the vaccines [165], yet the combination of inadequate information about long-term disease impacts and the promotion of vaccine-only strategies may be linked to skepticism about their role and to the attribution of harms to vaccines rather than the disease.
The Costs and the Benefits of Protective Measures
The costs of disease
A direct cost-benefit analysis shows that mitigating and suppressing COVID-19 is highly beneficial [166]. The costs of protective interventions and precautions, testing, isolation and quarantine support, surveillance, air cleaning measures etc. must be compared with ongoing costs of the uncontrolled spread of COVID-19 [149,167,168]. This includes all health, societal and economic consequences, short-term and long-term: symptomatic long COVID, organ and systemic damage, worsening of preexisting conditions, loss of quality of life [83], mental health impacts and cognitive deficits [84,169] orphanhood and loss of care [161], disability and death, labor force shortages and resulting supply chain disruptions [170]. The costs directly related to the disease alone are estimated in the billions even under the current conditions of deficient surveillance [168], while already in 2020, the impact of COVID-19 on the economy was estimated in the trillions [167]. According to a recent paper by Altmann et al. [171], “The oncoming burden of long COVID faced by patients, health-care providers, governments and economies is so large as to be unfathomable, which is possibly why minimal high-level planning is currently allocated to it.”
The costs of prevention in comparison to inaction
A key direction for action is indoor air sanitation. Clean air has great economic benefits even in non-pandemic times. A 2017 meta-analysis [172], reported that “The annual incremental energy and capital costs of increasing ventilation rates as needed to meet or exceed current standards, range from a few dollars to about ten dollars per person.” Citing a study from the U.S. Census Bureau data [173], the author added, “for reference, these costs can be compared to the US per student annual spending of $10.3K in 2009 for public elementary and secondary schools. Thus, the energy and capital costs of increasing ventilation rates would be less than 0.1% of education spending.” Mass testing and distribution of respirator masks are also very affordable and effective measures [166]. Such expenditures are negligible in comparison to the costs of endless COVID-19 infections. The economic benefits of a healthy population with a good quality of life are difficult to quantify, but the available estimates show that we cannot afford the ongoing costs of uncontrolled viral spread, while significantly reducing or even entirely stopping the spread is much more cost-effective. The benefit comparisons are also understandable in terms of historically clean water [174,175] and clean air [176,177].
The Plan for Action
Applying available measures to stop the uncontrolled spread
Achieving the goal of limiting the spread of COVID-19 is neither impossible nor a mystery. The primarily airborne transmission route of SARS-CoV-2 is no longer disputed [1]. To effectively combat the virus, we should develop a plan akin to the implementation of clean water to control cholera. The scientific consensus is clear [178,179]: we have the tools and strategies available to control the spread of COVID-19 through proactive, science-based interventions, and applying them is the only way to end the public health crisis. Available science and technology should be used to both to prevent airborne spread and to promptly identify infected individuals. Their implementation thus far has been inadequate, but it need not continue to be. We must promote, normalize, support and enforce measures which reduce airborne transmission by preventing the sharing of unfiltered air. The best source control is provided by fitted respirator masks [180]. In addition, key framework is bringing indoor air quality up to infection-control standards by monitoring CO2 and particles [181] and improving mechanical ventilation and filtration. Physical distancing or directed air flows protect also those who are unable to mask [182]. In addition, surveillance testing, tracking, wastewater analysis, organized and supported science-based isolation and quarantine, vaccination, and ongoing robust, targeted, scientifically honest information campaigns are essential. Random sample testing and emerging technology such as air sampling [183], electronic viral detectors [184], and highly effective trained dogs [185] can be used to monitor frequented areas and enhance case identification in healthcare, long-term care and other institutions. Finally, perhaps the most important measure is to provide the public with accurate information about the realistic long-term risks of infections, the nature of airborne transmission and effective protective measures.
Transitioning from uncontrolled spread to sustainable infection control
It is important to recognize that by using enhanced levels of some measures, we can reduce the need for others, compensating for limitations in other methods, or conditions in which it is difficult or impossible to implement them [186]. These methods and their strategic implementation have already been used during the pandemic with success and will be safe to reduce only once community transmission has been dramatically limited. Currently, there are high levels of transmission and inadequate clean air infrastructure, which means that additional intensive actions are necessary. However, as time goes on, it will be possible to limit these elevated precautions to areas with localized outbreaks. These actions should include normalizing the use of respirator masks, testing, supported isolation and quarantine, vaccination, and minimizing high-risk conditions by promoting remote work, which has already become widely accepted with about half working from home in many urban areas [187] and up to a third willing to quit or look for a new job if required to return to the employer’s worksite full-time [188]. In addition, remote work has numerous other important economic, health and environmental benefits [187]. It is not practical or necessary to implement all intensive measures in every context or location. In cases where one of these measures is not adopted, it may be effective to increase other measures, such as by using enhanced air filtering or directed air flows, higher frequency testing, and the use of high-quality respirators above the N95 standard. By slowing the spread, we can significantly reduce replication rates and thus the emergence of new variants. This will strengthen the effectiveness of updated vaccinations and treatments, improve conditions in healthcare systems, and protect our collective health. Once infection levels are brought down overall, localized contact tracing can stop further outbreaks and quarantining and testing contacts should be the standard international protocol to maintain sustainable control over viral spread. As is traditional with a highly contagious and harmful disease, adequate medically supervised isolation, either in person or through telemedicine [189] and at least one post-acute check-up can both help stop the spread and promptly and detect, report and treat resulting morbidity.
The role and responsibility of healthcare professionals and institutions
Healthcare institutions are by the nature of their responsibility to protect health [41,190-192] as well as the high levels of physical contact and concentrations of both vulnerable and COVID infected individuals—locations in which enhanced protections should be normalized. This includes multiple levels of precautions: the universal use of N95 or better respirators [193-196], high frequency testing, enhanced air hygiene, and optimized physical and social distancing. Some healthcare systems are already consistently implementing [197] or reintroducing such measures [198]. Educated healthcare workers are demanding the return of universal masking in healthcare settings [159,199]. Healthcare providers must adhere to their professional and ethical responsibility [200] to avoid causing harm by spreading COVID-19 and other airborne infections to patients and colleagues, or they may face not only medical, but also legal issues [201]. Professionals, including healthcare providers, should participate in coordinated local, regional and global action at the community, healthcare, and government levels. Healthcare settings, due to high risk conditions and responsibility for health, must set an example of good air hygiene and responsible masking behavior to protect their vulnerable patients and essential worker colleagues, and to encourage the general public to do the same.
Conclusion
The ongoing harm of COVID-19 has not been adequately acknowledged. The vascular, immune, neurological and other organ damage, persistent infection, chronic morbidity and disability due to infections and reinfections with SARS-CoV-2 are well established scientifically. This needs to be more widely communicated to the public and to healthcare providers. Generally unappreciated, this lack of communication leads to a wide discrepancy between historical risk taking and much higher current levels of risk and resulting harm. The costs of health and economic consequences of infections and reinfections dwarf those of available measures to control the spread and consequently also viral replication and new variant evolution. These measures include scientifically accurate public health messaging, instituting and implementing clean air policies, surveillance testing, normalizing masking, promoting safety in public spaces and work from home, and investing in research to develop sterilizing vaccines and treatments. Historical efforts in clean water, handwashing and disinfection in medical practice, building codes, automobile safety, HIV, and many others demonstrate that health and safety can be promoted by effective public communication, education and policy. The combination of acute disease, widespread symptomatic long COVID and pervasive organ damage point to the necessity for action to prevent ongoing transmission. It is important to recognize that feasibility of action is underestimated and costs and adverse social impacts of prevention are overestimated. Instead of disabling physical and mental health damage, practical approaches to “living with the virus”, mitigation, suppression and eventual elimination all require identical measures which are well known, available, affordable and feasible, and there is no reason to fail to apply them. A directional change to promote and preserve public health is feasible, worthwhile, and necessary.
References
1. Lewis, D. Why the WHO took two years to say COVID is airborne. Nature vol. 604 26–31 (2022).
2. Xu, S. wen, Ilyas, I. & Weng, J. ping. Endothelial dysfunction in COVID-19: an overview of evidence, biomarkers, mechanisms and potential therapies. Acta Pharmacologica Sinica vol. 44 695–709 (2023).
3. Z Varga, A. F. P. S. et al. Endothelial cell infection and endotheliitis in COVID-19. vol. 395 1417–1418 (Lancet Publishing Group, 2020).
4. Yang, R. C. et al. SARS-CoV-2 productively infects human brain microvascular endothelial cells. J. Neuroinflammation 19, 149 (2022).
5. Shen, X. R. et al. ACE2-independent infection of T lymphocytes by SARS-CoV-2. Signal Transduct. Target. Ther. 7, 1–11 (2022).
6. Maher, A. K. et al. Transcriptional reprogramming from innate immune functions to a pro-thrombotic signature by monocytes in COVID-19. Nat. Commun. 13, 1–17 (2022).
7. Ryan, F. J. et al. Long-term perturbation of the peripheral immune system months after SARS-CoV-2 infection. BMC Med. 20, (2022).
8. Bauer, L. et al. The neuroinvasiveness, neurotropism, and neurovirulence of SARS-CoV-2. Trends in Neurosciences vol. 45 358–368 (2022).
9. Emmi, A. et al. Detection of SARS-CoV-2 viral proteins and genomic sequences in human brainstem nuclei. npj Park. Dis. 9, 1–20 (2023).
10. Woo, M. S. et al. Vagus nerve inflammation contributes to dysautonomia in COVID-19. Acta Neuropathol. 146, 387–394 (2023).
11. Bussani, R. et al. Persistent SARS-CoV-2 infection in patients seemingly recovered from COVID-19. Journal of Pathology vol. 259 254–263 (John Wiley and Sons Ltd, 2023).
12. Proal, A. D. et al. SARS-CoV-2 reservoir in post-acute sequelae of COVID-19 (PASC). Nature Immunology 7 (2023) doi:10.1038/s41590-023-01601-2.
13. Chen, B., Julg, B., Mohandas, S. & Bradfute, S. B. Viral persistence, reactivation, and mechanisms of long COVID. eLife vol. 12 (2023).
14. Yang, C., Zhao, H., Espín, E. & Tebbutt, S. J. Association of SARS-CoV-2 infection and persistence with long COVID. The Lancet Respiratory Medicine vol. 11 504–506 (2023).
15. Bowe, B., Xie, Y. & Al-Aly, Z. Acute and postacute sequelae associated with SARS-CoV-2 reinfection. Nature Medicine vol. 28 2398–2405 (Nature Research, 2022).
16. Kovanen, P. T. & Vuorio, A. SARS-CoV-2 reinfection: Adding insult to dysfunctional endothelium in patients with atherosclerotic cardiovascular disease. Atherosclerosis Plus vol. 53 1–5 (2023).
17. Pinto Pereira, S. M. et al. Long COVID in Children and Young after Infection or Reinfection with the Omicron Variant: A Prospective Observational Study. J. Pediatr. 259, (2023).
18. Kuang, S. et al. Experiences of Canadians with long-term symptoms following COVID-19. Statistics Canada, Canadian COVID-19 Antibody and Health Survey – Follow-up Questionnaire (2023).
19. Ikeokwu, A. E. et al. Unveiling the Impact of COVID-19 Vaccines: A Meta-Analysis of Survival Rates Among Patients in the United States Based on Vaccination Status. Cureus 15, (2023).
20. Al-Aly, Z., Bowe, B. & Xie, Y. Long COVID after breakthrough SARS-CoV-2 infection. Nat. Med. 28, 1461–1467 (2022).
21. Ayoubkhani, D. et al. Risk of Long COVID in People Infected With Severe Acute Respiratory Syndrome Coronavirus 2 After 2 Doses of a Coronavirus Disease 2019 Vaccine: Community-Based, Matched Cohort Study. Open Forum Infect. Dis. 9, (2022).
22. Taquet, M., Dercon, Q. & Harrison, P. J. Six-month sequelae of post-vaccination SARS-CoV-2 infection: A retrospective cohort study of 10,024 breakthrough infections. Brain. Behav. Immun. 103, 154–162 (2022).
23. Van Egeren, D. et al. Vaccines Alone Cannot Slow the Evolution of SARS-CoV-2. Vaccines 11, 853 (2023).
24. Lopez-Leon, S. et al. Long-COVID in children and adolescents: a systematic review and meta-analyses. Sci. Rep. 12, 1–12 (2022).
25. Kompaniyets, L. et al. Post–COVID-19 Symptoms and Conditions Among Children and Adolescents — United States, March 1, 2020–January 31, 2022. MMWR. Morb. Mortal. Wkly. Rep. 71, 993–999 (2022).
26. Al-Aly, Z., Agarwal, A., Alwan, N. & Luyckx, V. A. Long COVID: long-term health outcomes and implications for policy and research. Nature Reviews Nephrology vol. 19 1–2 (2023).
27. Munipalli, B., Seim, L., Dawson, N. L., Knight, D. & Dabrh, A. M. A. Post-acute sequelae of COVID-19 (PASC): a meta-narrative review of pathophysiology, prevalence, and management. SN Compr. Clin. Med. 4, (2022).
28. Augustin, M. et al. Post-COVID syndrome in non-hospitalised patients with COVID-19: a longitudinal prospective cohort study. Lancet Reg. Heal. – Eur. 6, (2021).
29. Ben-Ami, R. et al. Epigenetic liquid biopsies reveal elevated vascular endothelial cell turnover and erythropoiesis in asymptomatic COVID-19 patients. bioRxiv 2023.07.28.550957 (2023) doi:10.1101/2023.07.28.550957.
30. Jacobson, K. B. et al. Patients With Uncomplicated Coronavirus Disease 2019 (COVID-19) Have Long-Term Persistent Symptoms and Functional Impairment Similar to Patients with Severe COVID-19: A Cautionary Tale During a Global Pandemic. Clin. Infect. Dis. 73, e826–e829 (2021).
31. Roessler, M. et al. Post-COVID-19-associated morbidity in children, adolescents, and adults: A matched cohort study including more than 157,000 individuals with COVID-19 in Germany. PLOS Med. 19, e1004122 (2022).
32. Ertesvåg, N. U. et al. Post COVID-19 condition after delta infection and omicron reinfection in children and adolescents. eBioMedicine 92, 104599 (2023).
33. Sirico, D. et al. Left ventricular longitudinal strain alterations in asymptomatic or mildly symptomatic paediatric patients with SARS-CoV-2 infection. European Heart Journal Cardiovascular Imaging vol. 23 1083–1089 (2022).
34. Heiss, R. et al. Pulmonary Dysfunction after Pediatric COVID-19. Radiology 306, (2023).
35. Dennis, A. et al. Multi-organ impairment and long COVID: a 1-year prospective, longitudinal cohort study. J. R. Soc. Med. 116, 97–112 (2023).
36. Parotto, M. et al. Post-acute sequelae of COVID-19: understanding and addressing the burden of multisystem manifestations. The Lancet. Respiratory medicine vol. 11 739–754 (2023).
37. Wu, X. et al. Damage to endothelial barriers and its contribution to long COVID. Angiogenesis (2023) doi:10.1007/s10456-023-09878-5.
38. Kalafatis, M. COVID-19: A Serious Vascular Disease with Primary Symptoms of a Respiratory Ailment. J. Appl. Lab. Med. 6, 1099–1104 (2021).
39. Ratchford, S. M. et al. Vascular alterations among young adults with SARS-CoV-2. Am. J. Physiol. – Hear. Circ. Physiol. 320, H404–H410 (2021).
40. Turner, S. et al. Long COVID: pathophysiological factors and abnormalities of coagulation. Trends in Endocrinology and Metabolism vol. 34 321–344 (2023).
41. Katsoularis, I. et al. Risks of deep vein thrombosis, pulmonary embolism, and bleeding after covid-19: nationwide self-controlled cases series and matched cohort study. BMJ (Clinical research ed.) vol. 377 e069590 (NLM (Medline), 2022).
42. Knight, R. et al. Association of COVID-19 With Major Arterial and Venous Thrombotic Diseases: A Population-Wide Cohort Study of 48 Million Adults in England and Wales. Circulation 146, 892–906 (2022).
43. Eberhardt, N. et al. SARS-CoV-2 infection triggers pro-atherogenic inflammatory responses in human coronary vessels. Nat. Cardiovasc. Res. 1–18 (2023) doi:10.1038/s44161-023-00336-5.
44. Podrug, M. et al. Long-Term Adverse Effects of Mild COVID-19 Disease on Arterial Stiffness, and Systemic and Central Hemodynamics: A Pre-Post Study. J. Clin. Med. 12, 2123 (2023).
45. Xie, Y., Xu, E., Bowe, B. & Al-Aly, Z. Long-term cardiovascular outcomes of COVID-19. Nature Medicine vol. 28 583–590 (Nature Research, 2022).
46. Roca-Fernandez, A. et al. Cardiac impairment in Long Covid 1-year post SARS-CoV-2 infection. Eur. Heart J. 43, (2022).
47. Sidik, S. M. Heart-disease risk soars after COVID – even with a mild case. Nature vol. 602 560 (2022).
48. Xu, E., Xie, Y. & Al-Aly, Z. Risks and burdens of incident dyslipidaemia in long COVID: a cohort study. Lancet Diabetes Endocrinol. 11, 120–128 (2023).
49. Zhang, V., Fisher, M., Hou, W., Zhang, L. & Duong, T. Q. Incidence of New-Onset Hypertension Post-COVID-19: Comparison With Influenza. Hypertens. (Dallas, Tex. 1979) 80, 2135–2148 (2023).
50. Peng, X. et al. Sharing CD4+ T Cell Loss: When COVID-19 and HIV Collide on Immune System. Frontiers in Immunology vol. 11 (2020).
51. Diao, B. et al. Reduction and Functional Exhaustion of T Cells in Patients With Coronavirus Disease 2019 (COVID-19). Front. Immunol. 11, 827 (2020).
52. Moriyama, M., Lucas, C., Monteiro, V. S. & Iwasaki, A. Enhanced inhibition of MHC-I expression by SARS-CoV-2 Omicron subvariants. Proc. Natl. Acad. Sci. U. S. A. 120, e2221652120 (2023).
53. Jing, Y. et al. SARS-CoV-2 infection causes immunodeficiency in recovered patients by downregulating CD19 expression in B cells via enhancing B-cell metabolism. Signal Transduct. Target. Ther. 6, 1–13 (2021).
54. Mao, W. et al. A methylation clock model of mild SARS‐CoV ‐2 infection provides insight into immune dysregulation . Mol. Syst. Biol. 19, e11361 (2023).
55. Ewing, A. COVID-19 and Immune Dysregulation, a Summary and Resource. WHN Sci. Commun. 4, 1–1 (2023).
56. Phetsouphanh, C. et al. Immunological dysfunction persists for 8 months following initial mild-to-moderate SARS-CoV-2 infection. Nature Immunology vol. 23 210–216 (Nature Research, 2022).
57. Wang, L. et al. Association of COVID-19 with respiratory syncytial virus (RSV) infections in children aged 0-5 years in the USA in 2022: A multicentre retrospective cohort study. Fam. Med. Community Heal. 11, 2456 (2023).
58. Kangabam, N. & Nethravathy, V. An overview of opportunistic fungal infections associated with COVID-19. 3 Biotech vol. 13 231 (2023).
59. Li, J. et al. Causal effects of COVID‐19 on cancer risk: A Mendelian randomization study. J. Med. Virol. 95, e28722 (2023).
60. Dugerdil, A., Semenzato, L., Weill, A., Zureik, M. & Flahault, A. Severe SARS-CoV-2 infection as a marker of undiagnosed cancer: a population-based study. Sci. Rep. 13, 1–12 (2023).
61. Jahankhani, K., Ahangari, F., Adcock, I. M. & Mortaz, E. Possible cancer-causing capacity of COVID-19: Is SARS-CoV-2 an oncogenic agent? Biochimie 213, 130–138 (2023).
62. Saini, G. & Aneja, R. Cancer as a prospective sequela of long COVID-19. BioEssays 43, (2021).
63. Rahimmanesh, I. et al. Cancer Occurrence as the Upcoming Complications of COVID-19. Frontiers in Molecular Biosciences vol. 8 813175 (2022).
64. Al-Aly, Z., Xie, Y. & Bowe, B. High-dimensional characterization of post-acute sequelae of COVID-19. Nature 594, 259–264 (2021).
65. Sharma, C. & Bayry, J. High risk of autoimmune diseases after COVID-19. Nature Reviews Rheumatology vol. 19 399–400 (2023).
66. Gracia-Ramos, A. E., Martin-Nares, E. & Hernández-Molina, G. New onset of autoimmune diseases following covid-19 diagnosis. Cells vol. 10 (2021).
67. Lim, S. H. et al. Autoimmune and Autoinflammatory Connective Tissue Disorders Following COVID-19. JAMA Netw. Open 6, e2336120 (2023).
68. Peng, K. et al. Risk of autoimmune diseases following COVID-19 and the potential protective effect from vaccination: a population-based cohort study. eClinicalMedicine 63, (2023).
69. Littlefield, K. M. et al. SARS-CoV-2-specific T cells associate with inflammation and reduced lung function in pulmonary post-acute sequalae of SARS-CoV-2. PLOS Pathog. 18, e1010359 (2022).
70. van Willigen, H. D. G. et al. One-Fourth of COVID-19 Patients Have an Impaired Pulmonary Function after 12 Months of Illness Onset. doi:10.2139/SSRN.4366836.
71. Bowe, B., Xie, Y., Xu, E. & Al-Aly, Z. Kidney Outcomes in Long COVID. J. Am. Soc. Nephrol. 32, 2851–2862 (2021).
72. Wanner, N. et al. Molecular consequences of SARS-CoV-2 liver tropism. Nat. Metab. 4, 310–319 (2022).
73. Zhang, T. et al. Risk for newly diagnosed diabetes after COVID-19: a systematic review and meta-analysis. BMC Medicine vol. 20 (2022).
74. Montefusco, L. et al. Acute and long-term disruption of glycometabolic control after SARS-CoV-2 infection. Nat. Metab. 3, 774–785 (2021).
75. Kendall, E. K., Olaker, V. R., Kaelber, D. C., Xu, R. & Davis, P. B. Association of SARS-CoV-2 Infection with New-Onset Type 1 Diabetes among Pediatric Patients from 2020 to 2021. JAMA Netw. Open 5, E2233014 (2022).
76. Ding, Q. & Zhao, H. Long-term effects of SARS-CoV-2 infection on human brain and memory. Cell Death Discov. 9, 1–8 (2023).
77. Petersen, M. et al. Brain imaging and neuropsychological assessment of individuals recovered from a mild to moderate SARS-CoV-2 infection. Proc. Natl. Acad. Sci. U. S. A. 120, (2023).
78. Douaud, G. et al. SARS-CoV-2 is associated with changes in brain structure in UK Biobank. Nature vol. 604 697–707 (Nature Research, 2022).
79. Guedj, E. et al. 18F-FDG brain PET hypometabolism in patients with long COVID. Eur. J. Nucl. Med. Mol. Imaging 48, 2823–2833 (2021).
80. Martínez-Mármol, R. et al. SARS-CoV-2 infection and viral fusogens cause neuronal and glial fusion that compromises neuronal activity. Sci. Adv. 9, (2023).
81. Dubey, S. et al. The Effects of SARS-CoV-2 Infection on the Cognitive Functioning of Patients with Pre-Existing Dementia. J. Alzheimer’s Dis. Reports 7, 119–128 (2023).
82. Di Primio, C. et al. Severe acute respiratory syndrome coronavirus 2 infection leads to Tau pathological signature in neurons. PNAS Nexus 2, (2023).
83. Walker, S. et al. Impact of fatigue as the primary determinant of functional limitations among patients with post-COVID-19 syndrome: a cross-sectional observational study. BMJ Open 13, e069217 (2023).
84. Hampshire, A. et al. Cognitive deficits in people who have recovered from COVID-19. eClinicalMedicine 39, 101044 (2021).
85. Fernández-Castañeda, A. et al. Mild respiratory COVID can cause multi-lineage neural cell and myelin dysregulation. Cell 185, 2452-2468.e16 (2022).
86. Cheetham, N. J. et al. The effects of COVID-19 on cognitive performance in a community-based cohort: a COVID symptom study biobank prospective cohort study. eClinicalMedicine 0, 102086 (2023).
87. Sauve, F. et al. Long-COVID cognitive impairments and reproductive hormone deficits in men may stem from GnRH neuronal death. eBioMedicine 96, (2023).
88. Taquet, M., Geddes, J. R., Husain, M., Luciano, S. & Harrison, P. J. 6-month neurological and psychiatric outcomes in 236 379 survivors of COVID-19: a retrospective cohort study using electronic health records. The Lancet Psychiatry 8, 416–427 (2021).
89. Xu, E., Xie, Y. & Al-Aly, Z. Long-term neurologic outcomes of COVID-19. Nature Medicine vol. 28 2406–2415 (Nature Research, 2022).
90. Bechmann, N. et al. COVID-19 Infections in Gonads: Consequences on Fertility? Hormone and Metabolic Research vol. 54 549–555 (2022).
91. Yang, M. et al. Pathological Findings in the Testes of COVID-19 Patients: Clinical Implications. Eur. Urol. Focus 6, 1124–1129 (2020).
92. Yamamoto, Y. et al. Detection of Male Hypogonadism in Patients with Post COVID‐19 Condition. J. Clin. Med. 11, 11 (2022).
93. Kaynar, M., Gomes, A. L. Q., Sokolakis, I. & Gül, M. Tip of the iceberg: erectile dysfunction and COVID-19. International Journal of Impotence Research vol. 34 152–157 (2022).
94. Aksak, T. et al. Investigation of the effect of COVID-19 on sperm count, motility, and morphology. J. Med. Virol. 94, 5201–5205 (2022).
95. Joshi, B. et al. The placental pathology in Coronavirus disease 2019 infected mothers and its impact on pregnancy outcome. Placenta 127, 1–7 (2022).
96. Jin, J. C. et al. SARS CoV-2 detected in neonatal stool remote from maternal COVID-19 during pregnancy. Pediatr. Res. 93, 1375–1382 (2023).
97. Stoecklein, S. et al. Effects of SARS-CoV-2 on prenatal lung growth assessed by fetal MRI. The Lancet Respiratory Medicine vol. 10 e36–e37 (2022).
98. Simon, E. et al. Impact of SARS-CoV-2 infection on risk of prematurity, birthweight and obstetric complications: A multivariate analysis from a nationwide, population-based retrospective cohort study. BJOG An Int. J. Obstet. Gynaecol. 129, 1084–1094 (2022).
99. Mongelli, A. et al. Evidence for biological age acceleration and telomere shortening in covid-19 survivors. Int. J. Mol. Sci. 22, 6151 (2021).
100. Guarnieri, J. W. et al. Core mitochondrial genes are down-regulated during SARS-CoV-2 infection of rodent and human hosts. Sci. Transl. Med. 15, (2023).
101. Gioia, U. et al. SARS-CoV-2 infection induces DNA damage, through CHK1 degradation and impaired 53BP1 recruitment, and cellular senescence. Nat. Cell Biol. 25, 550–564 (2023).
102. Kulasinghe, A. et al. Transcriptomic profiling of cardiac tissues from <scp>SARS‐CoV</scp> ‐2 patients identifies <scp>DNA</scp> damage. Immunology 168, 403–419 (2023).
103. Wang, R. et al. SARS-CoV-2 restructures host chromatin architecture. Nat. Microbiol. 8, 679–694 (2023).
104. Vojdani, A., Vojdani, E., Saidara, E. & Maes, M. Persistent SARS-CoV-2 Infection, EBV, HHV-6 and Other Factors May Contribute to Inflammation and Autoimmunity in Long COVID. Viruses vol. 15 (2023).
105. Stein, S. R. et al. SARS-CoV-2 infection and persistence in the human body and brain at autopsy. Nature 612, 758–763 (2022).
106. Natarajan, A. et al. Gastrointestinal symptoms and fecal shedding of SARS-CoV-2 RNA suggest prolonged gastrointestinal infection. Med 3, 371-387.e9 (2022).
107. Morens, D. M., Folkers, G. K. & Fauci, A. S. The Concept of Classical Herd Immunity May Not Apply to COVID-19. 226, 195–198 (2022).
108. Carabelli, A. M. et al. SARS-CoV-2 variant biology: immune escape, transmission and fitness. Nature Reviews Microbiology vol. 21 162–177 (2023).
109. Harvey, W. T. et al. SARS-CoV-2 variants, spike mutations and immune escape. Nature Reviews Microbiology vol. 19 409–424 (2021).
110. Uusküla, A. et al. Risk of SARS-CoV-2 infection and hospitalization in individuals with natural, vaccine-induced and hybrid immunity: a retrospective population-based cohort study from Estonia. Sci. Rep. 13, 20347 (2023).
111. Bobrovitz, N. et al. Protective effectiveness of previous SARS-CoV-2 infection and hybrid immunity against the omicron variant and severe disease: a systematic review and meta-regression. Lancet Infect. Dis. 23, 556–567 (2023).
112. Reynolds, C. J. et al. Immune boosting by B.1.1.529 (Omicron) depends on previous SARS-CoV-2 exposure. Science (80-. ). 377, (2022).
113. Breznik, J. A. et al. Early Omicron infection is associated with increased reinfection risk in older adults in long-term care and retirement facilities. eClinicalMedicine 63, 102148 (2023).
114. Nguyen, N. N., Houhamdi, L., Delorme, L., Colson, P. & Gautret, P. Reinfections with Different SARS-CoV-2 Omicron Subvariants, France. Emerg. Infect. Dis. 28, 2341–2343 (2022).
115. Focosi, D., Quiroga, R., McConnell, S., Johnson, M. C. & Casadevall, A. Convergent Evolution in SARS-CoV-2 Spike Creates a Variant Soup from Which New COVID-19 Waves Emerge. Int. J. Mol. Sci. 24, 2264 (2023).
116. Stanevich, O. V. et al. SARS-CoV-2 escape from cytotoxic T cells during long-term COVID-19. Nat. Commun. 14, 1–11 (2023).
117. Lane, G. et al. Quantity of SARS-CoV-2 RNA copies exhaled per minute during natural breathing over the course of COVID-19 infection. medRxiv doi:10.1101/2023.09.06.23295138.
118. Marschner, I. C. Estimating age-specific COVID-19 fatality risk and time to death by comparing population diagnosis and death patterns: Australian data. BMC Med. Res. Methodol. 21, 126 (2021).
119. Oseran, A. S. et al. Long term risk of death and readmission after hospital admission with covid-19 among older adults: retrospective cohort study. BMJ 382, (2023).
120. Amicone, M. et al. Mutation rate of SARS-CoV-2 and emergence of mutators during experimental evolution. Evol. Med. Public Heal. 10, 142–155 (2022).
121. Yang, Z. et al. Clinical Characteristics, Transmissibility, Pathogenicity, Susceptible Populations, and Re-infectivity of Prominent COVID-19 Variants. Aging and Disease vol. 13 402–422 (2022).
122. Hendrix, N., Sidky, H. & Sahner, D. K. Title: Influence of Prior SARS-CoV-2 Infection on COVID-19 Severity: Evidence from the National COVID Cohort Collaborative Full name, department, institution, city, and country of authors. medRxiv 2023.08.03.23293612 (2023) doi:10.1093/jamia/ocaa196.
123. Brazil, R. How your first brush with COVID warps your immunity. Nature vol. 613 428–430 (2023).
124. Nguyen, N. N., Nguyen, Y. N., Hoang, V. T., Million, M. & Gautret, P. SARS-CoV-2 Reinfection and Severity of the Disease: A Systematic Review and Meta-Analysis. Viruses 15, 967 (2023).
125. Tenforde, M. W. & Link-Gelles, R. Reduction in COVID-19-related mortality over time but disparities across population subgroups. The Lancet Public Health vol. 8 e327–e328 (2023).
126. FastStats – Leading Causes of Death. https://www.cdc.gov/nchs/fastats/leading-causes-of-death.htm.
127. Xie, Y., Choi, T. & Al-Aly, Z. Risk of Death in Patients Hospitalized for COVID-19 vs Seasonal Influenza in Fall-Winter 2022-2023. JAMA 329, 1697–1699 (2023).
128. Menegale, F. et al. Evaluation of Waning of SARS-CoV-2 Vaccine-Induced Immunity: A Systematic Review and Meta-analysis. JAMA Netw. Open 6, E2310650 (2023).
129. Ferdinands, J. M. et al. Waning of vaccine effectiveness against moderate and severe covid-19 among adults in the US from the VISION network: Test negative, case-control study. BMJ 379, (2022).
130. Huang, G. et al. The effect of the COVID-19 pandemic on life expectancy in 27 countries. Sci. Rep. 13, 1–11 (2023).
131. Davis, H. E., McCorkell, L., Vogel, J. M. & Topol, E. J. Long COVID: major findings, mechanisms and recommendations. Nature Reviews Microbiology vol. 21 133–146 (2023).
132. Marra, A. R. et al. Risk factors for long coronavirus disease 2019 (long COVID) among healthcare personnel, Brazil, 2020-2022. Infect. Control Hosp. Epidemiol. 1–7 (2023) doi:10.1017/ice.2023.95.
133. Waters, A. Long covid: nearly half of doctors affected can no longer work full time, finds survey. BMJ 382, 1529 (2023).
134. Up the line to death: covid-19 has revealed a mortal betrayal of the world’s healthcare workers – The BMJ. https://blogs.bmj.com/bmj/2021/01/29/up-the-line-to-death-covid-19-has-revealed-a-mortal-betrayal-of-the-worlds-healthcare-workers/.
135. First major survey of doctors with Long Covid reveals debilitating impact on health, life and work – BMA media centre – BMA. https://www.bma.org.uk/bma-media-centre/first-major-survey-of-doctors-with-long-covid-reveals-debilitating-impact-on-health-life-and-work-and-wider-implications-for-workforce-and-health-services.
136. Norredam, M., Hayward, S., Deal, A., Agyemang, C. & Hargreaves, S. Understanding and addressing long-COVID among migrants and ethnic minorities in Europe. The Lancet Regional Health – Europe vol. 19 100427 (2022).
137. Heller, O. et al. Prevalence of Long-COVID Among Low-Income and Marginalized Groups: Evidence From Israel. Int. J. Public Health 67, (2022).
138. Subramanian, A. et al. Symptoms and risk factors for long COVID in non-hospitalized adults. Nat. Med. 28, 1706–1714 (2022).
139. Bai, F. et al. Female gender is associated with long COVID syndrome: a prospective cohort study. Clin. Microbiol. Infect. 28, 611.e9-611.e16 (2022).
140. Sugiyama, A. et al. Long COVID occurrence in COVID-19 survivors. Sci. Rep. 12, 1–11 (2022).
141. Duval, D. et al. Long distance airborne transmission of SARS-CoV-2: rapid systematic review. BMJ 377, (2022).
142. Peluso, M. J. et al. Title: Multimodal Molecular Imaging Reveals Tissue-Based T Cell Activation and Viral RNA 1 Persistence for Up to 2 Years Following COVID-19 2 3 Short Title: Tissue T Cell Activation and Viral RNA Persistence Following COVID-19 4 5. doi:10.1101/2023.07.27.23293177.
143. Yeh, K. B. et al. Significance of High-Containment Biological Laboratories Performing Work During the COVID-19 Pandemic: Biosafety Level-3 and -4 Labs. Front. Bioeng. Biotechnol. 9, (2021).
144. Demoliou, C., Papaneophytou, C. & Nicolaidou, V. SARS-CoV-2 and HIV-1: So Different yet so Alike. Immune Response at the Cellular and Molecular Level. Int. J. Med. Sci. 19, 1787–1795 (2022).
145. Li, D., Jiang, S. F., Lei, N. Y., Shah, S. C. & Corley, D. A. Effect of Helicobacter pylori Eradication Therapy on the Incidence of Noncardia Gastric Adenocarcinoma in a Large Diverse Population in the United States. Gastroenterology 165, 391-401.e2 (2023).
146. AIDS and COVID-19 are two diseases separated by a common lymphocytopenia. https://www.fortunejournals.com/articles/aids-and-covid19-are-two-diseases-separated-by-a-common-lymphocytopenia.html.
147. Pan, T. et al. A single-cell atlas reveals shared and distinct immune responses and metabolic profiles in SARS-CoV-2 and HIV-1 infections. Front. Genet. 14, (2023).
148. Agbuduwe, C. & Basu, S. Haematological manifestations of COVID-19: From cytopenia to coagulopathy. Eur. J. Haematol. 105, 540–546 (2020).
149. New data shows long Covid is keeping as many as 4 million people out of work | Brookings. https://www.brookings.edu/articles/new-data-shows-long-covid-is-keeping-as-many-as-4-million-people-out-of-work/.
150. Tartof, S. Y. et al. Health Care Utilization in the 6 Months Following SARS-CoV-2 Infection. JAMA Netw. Open 5, E2225657 (2022).
151. Long COVID – Household Pulse Survey – COVID-19. https://www.cdc.gov/nchs/covid19/pulse/long-covid.htm.
152. Sørensen, A. I. V. et al. A nationwide questionnaire study of post-acute symptoms and health problems after SARS-CoV-2 infection in Denmark. Nat. Commun. 13, 1–8 (2022).
153. Seeßle, J. et al. Persistent Symptoms in Adult Patients 1 Year After Coronavirus Disease 2019 (COVID-19): A Prospective Cohort Study. Clin. Infect. Dis. 74, 1191–1198 (2022).
154. Alkodaymi, M. S. et al. Prevalence of post-acute COVID-19 syndrome symptoms at different follow-up periods: a systematic review and meta-analysis. Clinical Microbiology and Infection vol. 28 657–666 (2022).
155. Zhao, Y. et al. The phenotype and prediction of long-term physical, mental and cognitive COVID-19 sequelae 20 months after recovery, a community-based cohort study in China. Mol. Psychiatry 28, 1793–1801 (2023).
156. Van Wambeke, E. et al. Two-Years Follow-Up of Symptoms and Return to Work in Complex Post-COVID-19 Patients. J. Clin. Med. 12, 741 (2023).
157. Orban, Z. S., Visvabharathy, L., Perez Giraldo, G. S., Jimenez, M. & Koralnik, I. J. SARS-CoV-2-Specific Immune Responses in Patients With Postviral Syndrome After Suspected COVID-19. Neurol. Neuroimmunol. neuroinflammation 10, (2023).
158. Au, L., Capotescu, C., Eyal, G. & Finestone, G. Long covid and medical gaslighting: Dismissal, delayed diagnosis, and deferred treatment. SSM – Qual. Res. Heal. 2, 100167 (2022).
159. Kalu, I. C., Henderson, D. K., Weber, D. J. & Haessler, S. Back to the future: Redefining universal precautions to include masking for all patient encounters. Infection Control and Hospital Epidemiology vol. 44 1373–1374 (2023).
160. Stephenson, T. et al. Physical and mental health 3 months after SARS-CoV-2 infection (long COVID) among adolescents in England (CLoCk): a national matched cohort study. Lancet. Child Adolesc. Heal. 6, 230–239 (2022).
161. Cox, D. What will happen to the orphans of covid-19? BMJ 379, (2022).
162. Taquet, M. et al. Neurological and psychiatric risk trajectories after SARS-CoV-2 infection: an analysis of 2-year retrospective cohort studies including 1 284 437 patients. The Lancet Psychiatry 9, 815–827 (2022).
163. Sule, S. et al. Communication of COVID-19 Misinformation on Social Media by Physicians in the US + Supplemental content. JAMA Netw. Open 6, 2328928 (2023).
164. Tseng, Y. J., Olson, K. L., Bloch, D. & Mandl, K. D. Smart Thermometer-Based Participatory Surveillance to Discern the Role of Children in Household Viral Transmission During the COVID-19 Pandemic. JAMA Netw. Open 6, E2316190 (2023).
165. Lisman, D. et al. Molecular Diagnosis of COVID-19 Sudden and Unexplained Deaths: The Insidious Face of the Pandemic. Diagnostics 13, 2980 (2023).
166. Philippe, C. et al. Mass testing to end the COVID-19 public health threat. The Lancet Regional Health – Europe vol. 25 100574 (2023).
167. Cutler, D. M. & Summers, L. H. The COVID-19 Pandemic and the $16 Trillion Virus. JAMA – Journal of the American Medical Association vol. 324 1495–1496 (2020).
168. The Economic Cost of Long COVID: An Update – David Cutler | Harvard Kennedy School. https://www.hks.harvard.edu/centers/mrcbg/programs/growthpolicy/economic-cost-long-covid-update-david-cutler.
169. Premraj, L. et al. Mid and long-term neurological and neuropsychiatric manifestations of post-COVID-19 syndrome: A meta-analysis. Journal of the Neurological Sciences vol. 434 (2022).
170. Goda, G. S. & Soltas, E. The Impacts of Covid-19 Illnesses on Workers. (2022) doi:10.3386/w30435.
171. Altmann, D. M., Whettlock, E. M., Liu, S., Arachchillage, D. J. & Boyton, R. J. The immunology of long COVID. Nature Reviews Immunology vol. 23 618–634 (2023).
172. Fisk, W. J. The ventilation problem in schools: literature review. Indoor Air 27, 1039–1051 (2017).
173. Statistical Abstract of the United States: 2012. https://www.census.gov/library/publications/2011/compendia/statab/131ed.html.
174. Hutton, G., Haller, L. & Bartram, J. Global cost-benefit analysis of water supply and sanitation interventions. J. Water Health 5, 481–501 (2007).
175. Bring trillions of dollars to developing country economies by making clean water, decent toilets and hygiene available for all - report | WaterAid Global. https://www.wateraid.org/media/bring-trillions-of-dollars-to-developing-country-economies-with-clean-water.
176. Benefits and Costs of the Clean Air Act | Overview of the Clean Air Act and Air Pollution | US EPA. https://19january2021snapshot.epa.gov/clean-air-act-overview/benefits-and-costs-clean-air-act_.html.
177. Benefits and Costs of the Clean Air Act 1990-2020, the Second Prospective Study | US EPA. https://www.epa.gov/clean-air-act-overview/benefits-and-costs-clean-air-act-1990-2020-second-prospective-study.
178. Lazarus, J. V. et al. A multinational Delphi consensus to end the COVID-19 public health threat. Nature 611, 332–345 (2022).
179. Flahault, A. et al. No time for complacency on COVID-19 in Europe. The Lancet vol. 401 1909–1912 (2023).
180. Blachere, F. M. et al. Face mask fit modifications that improve source control performance. Am. J. Infect. Control 50, 133–140 (2022).
181. Park, S. & Song, D. CO2 concentration as an indicator of indoor ventilation performance to control airborne transmission of SARS-CoV-2. J. Infect. Public Health 16, 1037–1044 (2023).
182. Ferrari, S. et al. Ventilation strategies to reduce airborne transmission of viruses in classrooms: A systematic review of scientific literature. Building and Environment vol. 222 109366 (2022).
183. Temte, J. et al. Four Methods for Monitoring SARS-CoV-2 and Influenza A Virus Activity in Schools. JAMA Netw. Open 6, e2346329 (2023).
184. Monteil, S., Casson, A. J. & Jones, S. T. Electronic and electrochemical viral detection for point-of-care use: A systematic review. PLoS ONE vol. 16 (2021).
185. Kantele, A. et al. Scent dogs in detection of COVID-19: triple-blinded randomised trial and operational real-life screening in airport setting. BMJ Glob. Heal. 7, 8024 (2022).
186. Frosch, R. A. Notes toward a theory of the management of vulnerability. in Seeds of Disaster, Roots of Response: How Private Action Can Reduce Public Vulnerability 77–98 (Cambridge University Press, 2006). doi:10.1017/CBO9780511509735.009.
187. Work From Home: A 21st Century Revolution – WHN. https://whn.global/newsletter/work-from-home-revolution/?emci=07984cbe-768d-ee11-8924-6045bdd47111&emdi=414573b0-108e-ee11-8924-6045bdd47111&ceid=14462724.
188. Giray, C. et al. Working from home around the world. (2023).
189. Boucau, J. et al. Duration of Shedding of Culturable Virus in SARS-CoV-2 Omicron (BA.1) Infection. N. Engl. J. Med. 387, 275–277 (2022).
190. Doctor Patient Relationship. https://www.bma.org.uk/advice-and-support/ethics/doctor-patient-relationship/doctor-patient-relationship.
191. Dagher, H. et al. Long COVID in cancer patients: preponderance of symptoms in majority of patients over long time period. Elife 12, (2023).
192. Hatfield, K. M. et al. Assessment of Hospital-Onset SARS-CoV-2 Infection Rates and Testing Practices in the US, 2020-2022. JAMA Netw. open 6, e2329441 (2023).
193. Szanyi, J., Howe, S., Wilson, T. & Blakely, T. Consistent mask use and SARS-CoV-2 epidemiology: a simulation modelling study. Medical Journal of Australia vol. 219 77–79 (2023).
194. Heiman, S. L. et al. Descriptive norms caused increases in mask wearing during the COVID-19 pandemic. Sci. Rep. 13, 11856 (2023).
195. Snyder, G. M., Passaretti, C. L. & Stevens, M. P. Hospital approaches to universal masking after public health unmasking guidance. Infect. Control Hosp. Epidemiol. 10, 845–846 (2023).
196. Leech, G. et al. Mask wearing in community settings reduces SARS-CoV-2 transmission. Proc. Natl. Acad. Sci. U. S. A. 119, e2119266119 (2022).
197. Ensuring Your Safety At Our Facilites | RWJBarnabas Health. https://www.rwjbh.org/patients-visitors/what-you-need-to-know-about-covid-19/ensuring-your-safety-at-our-facilities/.
198. Several hospitals tighten mask rules | CBC News. https://www.cbc.ca/news/canada/ottawa/ottawa-kingston-hospital-mask-rule-covid-flu-1.6959762.
199. Christie, B. Covid-19: Bring back mandatory mask wearing in health settings, say Scottish workers. BMJ 382, p1648 (2023).
200. Dorfman, D., Raz, M. & Berger, Z. Physicians’ Refusal to Wear Masks to Protect Vulnerable Patients—An Ethical Dilemma for the Medical Profession. JAMA Heal. Forum 4, e233780 (2023).
201. Hospitals That Ditch Masks Risk Exposure – Bill of Health. https://blog.petrieflom.law.harvard.edu/2023/02/20/hospital-liability-covid-infection/.